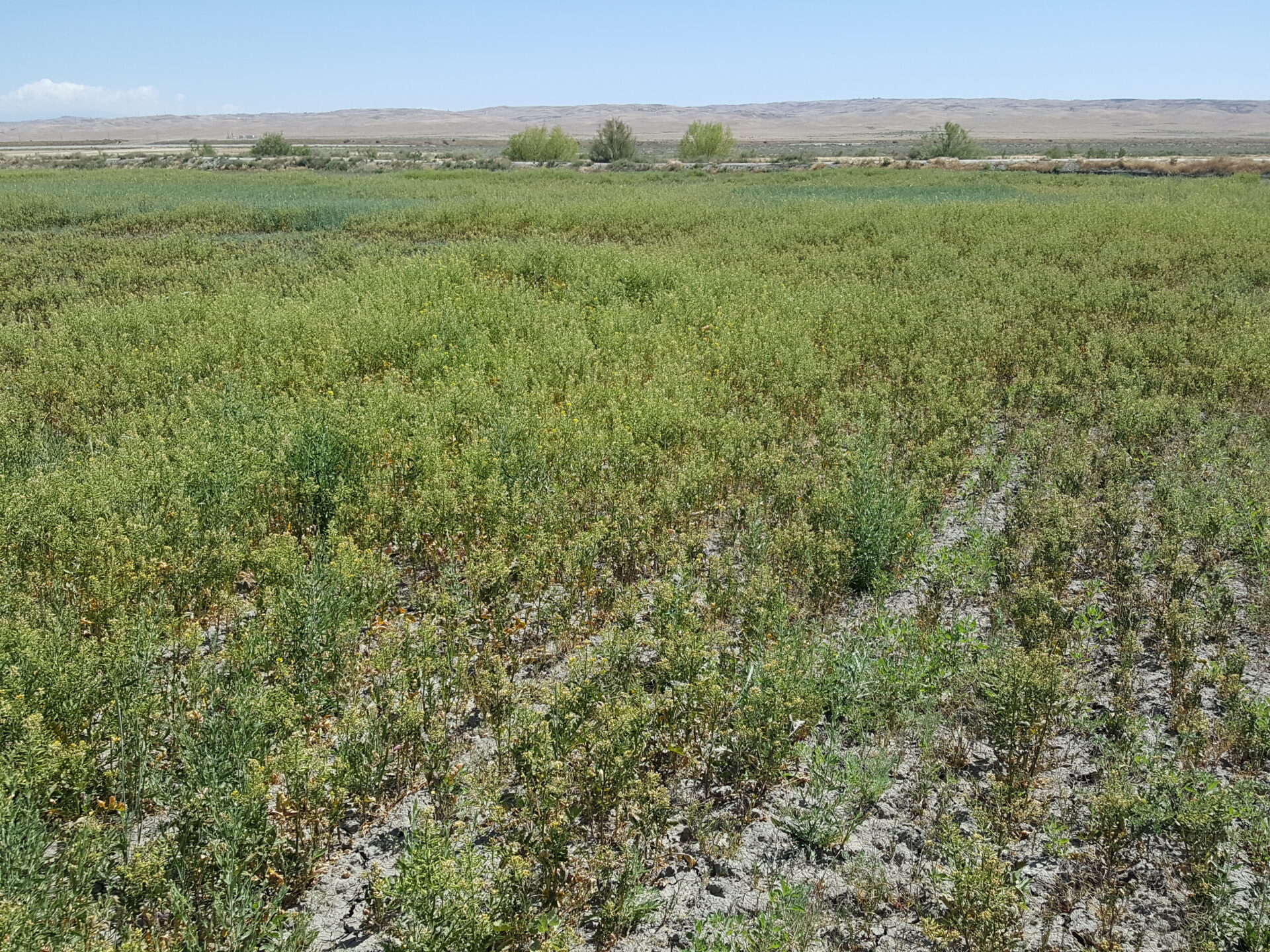
The presence of excess salts in the ground is a far-reaching and expanding threat to agriculture across the globe. Increases in soil salinity are considered to be the primary stress to global crop production (Laidero 2012). According to the Food and Agriculture Organization of the United Nations, 1% to 2% of all irrigated acreage is taken out of production every year due to excessive salt loads. Addressing these issues before it is too late has become imperative to maintaining the continued productivity of certain regions.
The destruction of arable land has had some profound and lasting social and economic effects. In their Assessment Report on Land Degradation and Restoration, the Intergovernmental Science-Policy Platform on Biodiversity and Ecosystem Service (IPBES) says that 190 million acres of primarily irrigated land have been permanently lost to salinity. Furthermore, there are currently 150 million acres of arable land damaged by salinization (Montanarella et al. 2018). Within the U.S., the most affected areas are located in the arid western portion of the country. The Colorado River Basin, which includes parts of California, Colorado, Arizona, Nevada, New Mexico, Utah and Wyoming, has seen particularly concentrated effects (LaHue 2017).
Cadillac Desert, by Marc Reisner (1993), provides a warning to all who plough forward untempered into the American desert:
Desert, semidesert, call it what you will. The point is that despite heroic efforts and many billions of dollars, all we have managed to do in the arid west is turn a Missouri-size section green – and that conversion has been wrought mainly with nonrenewable groundwater. But a goal of many … has long been to double, triple, quadruple the amount of desert that has been civilized and farmed, and now these same people say that the future of a hungry world depends on it, even if it means importing water from as far away as Alaska. What they seem not to understand is how difficult it will be just to hang on to the beachhead they have made. Such a surfeit of ambition stems, of course, from the remarkable record of success we have had in reclaiming the American desert. But the same could have been said about any number of desert civilizations throughout history – Assyria, Carthage, Mesopotamia; the Inca, the Aztec, the Hohokam – before they collapsed. And it may not have even been drought that did them in. It may have been salt.
Arid and semi-arid environments are most at-risk due to a dependence on irrigation water that tends to contain higher levels of salt. Once on the surface, water evaporates, leaving salts behind. Notice the correlation between arid regions of the world and the distribution of saline, sodic and saline-sodic soils in Figure 1.
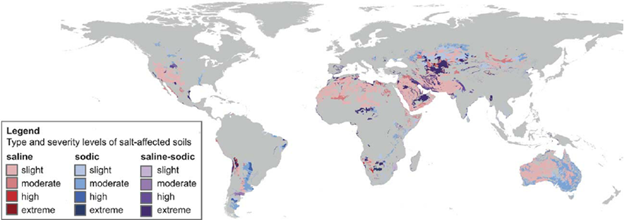
It doesn’t take many years for salt deposits to build up to levels that are toxic to many species of plants. Soils and plants impacted by excess salts can exhibit detrimental effects on their physical, chemical and biological properties. Land-use options and productivity are adversely affected by excess salts, which can lead to drops in productivity and property value. Depending on the amount and type of salt in the soil, impacts will differ (McCauley and Jones 2005).
Sources and Causes
The vast majority of salts come from the natural weathering of parent material, which includes minerals from bedrock and ancient sea beds (Cardon et al. 2007). Water-soluble salts are flushed from the parent material and flow downward into subterranean water basins, which are a primary source of irrigation water. Other sources of salts include damming of rivers, excessive use of agricultural fertilizers, municipal runoff and water treatment with “softeners”. In coastal areas, excessive pumping of groundwater can create intrusion zones where salty sea water penetrates freshwater aquifers. Sea water intrusion of groundwater pumping sites occurs most prominently when there is insufficient groundwater recharge from rain and rivers to offset the amount being pumped out.
Arid and semi-arid regions are characterized in part by their limited annual precipitation. During dry periods, groundwater recharge slows and pumping and evaporation from the soil increases. These combined factors cause groundwater levels to drop and salt deposition on the soil surface to increase. Droughts in these regions further exacerbate the issue. To take an example from California’s Central Valley, “…salt in the San Joaquin Valley continues to increase, especially during drought years. That’s because during droughts, California’s farms and cities rely on groundwater for up to 60% of their freshwater supply, up from 35% in non-drought years, and groundwater tends to be saltier than river water. People have been using groundwater faster than it naturally replenishes, dropping water levels deeper underground” (Gies 2017).
Salinity Impact on Plant Growth and Yield
Stress in the form of salinity is the most limiting environmental factor affecting plant growth in regions where rainfall is limited (Parida and Das 2005). Salts limit plant growth via several pathways. First, saline soils reduce a plant’s ability to absorb water. “Osmotic stress symptoms are very similar to those of drought stress, and include stunted growth, poor germination, leaf burn, wilting and possibly death” (McCauley and Jones, 2005). These symptoms, similar to drought stress, occur even when water is present in the soil. In addition to affecting a plant’s ability to take up water, excess salinity can affect nutrient availability and uptake, and it can cause toxicity issues from sodium and chlorine (Evelin et al. 2009).
The effects that salt have on plants vary depending on the type of crop being grown, the amount of salt, and the type of salts in the soil. The presence of salts in the shallow layers of the soil profile will have a greater negative impact than those at layers further down in the profile due to their proximity to plant roots.
Salt tolerance varies greatly from crop to crop. Carrots and strawberries, for example, are sensitive enough to suffer yield and growth losses in soils considered to be “very slightly saline,” while asparagus and chard are tolerant to much higher levels of salts. Each crop species has a corresponding level of tolerance to salinity, and beyond this level, growth and yield begin to diminish (see Table 1 on page 18, for more information on crop-specific tolerance levels.) Many plants will not display negative effects of salinity stress; thus, observational analysis may not be sufficient to determine if salts are affecting the yield of a particular crop. In order to be certain and establish an appropriate management plan, soil and irrigation water testing are essential.
Example from Table 1: Sweet potato grown in soil where EC measures 3.8 can be expected to yield around 75% of maximum. Note: These numbers represent rough guidelines that will be influenced by a number of factors, including cultivar chosen, soil temperature, cultural practices and use of rootstocks, to name a few.
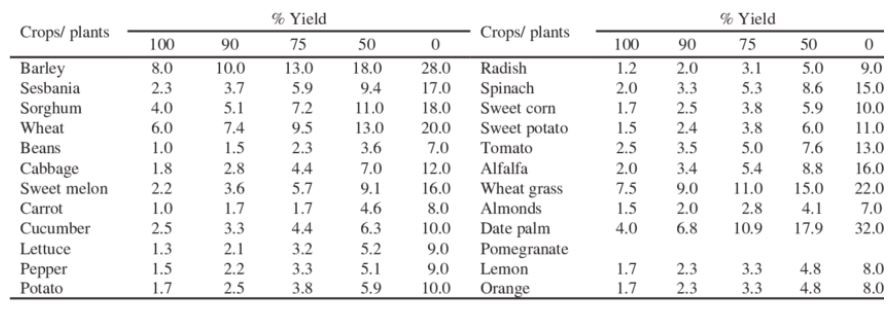
Salinity and the Soil
Salinity and sodicity are the two salt-related problems that impact land managers around the globe. They are similar in many of their characteristics but should be managed differently due to the chemical differences in their composition. Saline-sodic soil is the third possibility. These soils exhibit traits of both types and require a management approach similar to that for sodic soils.
The most common salts include sodium (Na+ ), magnesium (Mg2+) and calcium (Ca2+ ). Other salts present to a lesser extent include potassium (K+), chloride (Cl-), bicarbonate (HCO3-) and sulfate (SO42-).
Soil structure is one of the fundamental aspects of a soil that can help us understand whether it is functioning properly. Soils are composed of varying proportions of sand, silt and clay. Structure relates to the way these particles aggregate, or clump together, on a chemical level. Well aggregated soils allow for healthy soil function, which includes the soil’s ability to circulate air and percolate water down into the profile. Changes observed in soil structure or function may be indicative of certain salinity issues.
Soils characterized as saline or saline-sodic can be a wolf in sheep’s clothing because they can appear to have good structure while negatively impacting other biological and chemical properties. Sodicity, on the other hand, is a specific salinity issue in which excess sodium can contribute to the breakdown of soil structure. Sodic soils will facilitate the development of surface crusts, which hamper the germination and emergence of seedlings. These crusts form dense layers that inhibit root growth and make tillage more difficult. The destruction of soil aggregates also reduces pore structure and causes a settling of soil particles that are loosely or not at all associated to their neighboring particles (Abrol et al. 1988).
The physical structure of sodic soils, as described above, also leads to topsoil that is highly susceptible to the erosive forces of wind and water. Another distinguishing characteristic of sodic soils is that they have a high pH, usually 8.5 or higher. It is the tendency of high-pH soils to decrease the availability of essential nutrients, including calcium, magnesium, phosphorus, potassium, iron, manganese and zinc, which is cause for concern.
Remediating Saline Soils
Aside from a few very expensive management options, flushing salts further down into the soil profile with clean (low-salt) water is the only way to directly lower salt concentrations in managed soils. Limiting the use of inputs that have high concentrations of salt, such as saline irrigation water, chemical fertilizers or dairy manure, will serve as a good first step in reducing salt loads. Because good drainage is important to the leaching of salts, employing soil-management practices that improve soil structure and hydraulic flow through your farm’s soil will aid in moving salts away from sensitive crop-growing areas.

Measuring Salinity and Sodicity
Three factors typically examined in order to determine a soils classification in terms of salinity are Electrical Conductivity (EC), Sodium Absorption Ratio (SAR) and pH.
Electrical Conductivity (EC), sometimes referred to as specific conductance, measures the ease with which current can pass through an object. In this case, the object is the soil (ECe) or irrigation water (ECw). The more salt in a sample, the more easily a current will pass through that sample. Lab test results will provide measurements in the form of millimhos per cm (mmhos/cm) or decisiemens per meter (dS/m). These units are equivalent to one another: 1 mmho/cm = 1 dS/m.
The most reliable test for sodicity of soil or water is the sodium absorption ratio (SAR), which compares the concentration of sodium to calcium and magnesium. An alternate test that is also used to determine sodicity is the exchangeable sodium percentage (ESP). This test compares the amount of sodium relative to the cation exchange capacity (CEC) of the soil sample. CEC is a term that describes the ability of a soil particle (negatively charged) to bind to positively charged molecules or elements like salt (Hazelton and Murphy 2016).
Remediating Sodic and Saline-Sodic Soils
Due to low permeability, soils classified as saline-sodic or sodic require an additional step in order to effectively flush salts down into the soil profile while maintaining or improving soil function. Some form of calcium, usually gypsum, is used to replace excess sodium present in the soil. Due to its stronger charge, calcium can replace the sodium attached to soil particles. Freed sodium then converts to salt in the form of Na2SO4, which is more easily leached from the soil. Some organic amendments have been shown to be excellent options when remediating alkaline soils (sodic soils tend to be alkaline). Whether biochar, biosolids, compost or green waste compost is applied, each will reduce EC, ESP and SAR to varying degrees, and when combined with gypsum, will reduce salinity to a greater degree than gypsum or organic components alone (Chaganti et al. 2015).
Management for Soil Remediation
Particular management practices and related factors can increase the likelihood that salts will deposit on the soil surface. Poor management of irrigation water and excess tillage can exacerbate salinity problems. Excessive tillage, for example, can create a hardpan under the soil surface. This is a layer through which water percolates very slowly or not at all. Also, saline water sitting just below the soil surface can rise to the surface through capillary action. Capillary action describes the ability of water to move through narrow spaces regardless of external forces, including gravity. If you have ever left a paper towel to soak up water and watched the water spread across its fibers, you were observing capillary action. This is the same process that occurs in the soil when water is able to migrate upward through the soil profile. In this case, the water, with its load of salt, migrates to the surface, and as the water evaporates continually, it leaves ever-increasing amounts of salt behind.
The above-listed remediation techniques will do little to improve soil salinity conditions when hardpan layers or excessive soil compaction are present on the farm. In these situations, it may be advisable to break up impermeable layers mechanically (“ripping” the soil with deep ploughing or subsoiler) followed by a reduction in soil management practices that create these adverse conditions (Abrol et al. 1988). The reduction in salinity-enhancing management practices must be accompanied by implementation of practices that can help mitigate saline conditions.
Role of Organic Matter
Adding organic amendments is a viable method of addressing the negative impacts of salinity in the soil. Saline soils have been shown to benefit from compost, manure, green wastes and other organic amendments, which reduce the impact of erosive forces and improve soil structure and soil function (Diacono and Montemurro 2015). Additionally, organic matter aids in jump-starting biological and chemical processes, which can buffer the negative effects imposed by salt and help to increase nutrient cycling and availability (Rao and Pathak 1996).
Adding organic matter helps maintain the soil ecosystem, which can improve soil physical properties through the stabilization of aggregates. Bacteria and fungi release various glue-like substances through their metabolic processes that contribute to the adhesion of soil particles, creating soil aggregates. Increases in organic matter in soils impacted by excess salts have been shown to increase soil porosity and aeration (organic matter has much the same effect in non-saline soils), resulting in greater infiltration rates and reduction in soil salt content when flushed with water that is low in soluble salts (Diacono and Montemurro 2015).
In other experiments, under saline conditions, the addition of poultry manure and compost has been shown to increase available potassium. The addition of soluble and exchangeable potassium (K+) acts in a similar capacity to calcium and magnesium; that is to say, under sodic conditions, potassium will compete with sodium for space on the soil particles. What’s more, K plays an important role in the physiological function of plants, which can buffer some of the detrimental effects of salt stress (Diacono and Montemurro 2015).
Mulching is another practice that effectively limits the amount of salt accumulation on the soil surface because it reduces evaporation from the soil surface by 50% to 80%. Mulches can take various forms and can be used in a number of ways. Plastic cover and organic mulches are both effective options. Although both plastic and organic mulches are viable options for the reduction of salt accumulation, there are associated costs and benefits to both. Plastic mulches may require lower management and labor cost than organic mulches, but they are less effective than organic mulches when it comes to reducing salt accumulation (on average, 32% less salt accumulation on organic mulches) (Aragues et al. 2014). Plastic mulches can intercept precipitation, which reduces any potential leaching effect from rainfall. Plastic mulches also deteriorate over time and require disposal (Aragues et al. 2014). Organic mulches, on the other hand, may decompose rapidly and need to be replaced more often. They also benefit the soil ecosystem by feeding it and promoting earthworm populations (Jodaugiene et al. 2010).
Concluding Thoughts
American agriculture is facing increasing challenges and experiencing severe climate events more often. Farmers are having to deal with unprecedented droughts, flooding, and fires, as well as more erratic and extreme precipitation and temperatures. If we are to avoid the fates of the civilizations Marc Reisner noted in the introduction—Assyria, Carthage, Mesopotamia, the Inca, the Aztec, the Hohokam—we, as a society, must pay more attention to the way we manage our soils and learn to treat them as the complex ecosystems that they are. Fortunately, we have more knowledge than ever before about how soils should be managed for soil health, and about the impacts of irrigation in arid environments.
Resources
To learn more about related topics, please visit the ATTRA website (www.attra.ncat.org)
If you would like to learn more about testing for salinity, salt mitigating management practices and a complete resource list, please check out the full publication Saline and Sodic Soils: Identification, Mitigation, and Management Considerations at attra.ncat.org/product/saline-and-sodic-soils-identification-mitigation-and-management-considerations/.
Practices that increase soil health and promote beneficial microbial species can mitigate many of the challenges presented by salty soils. Management practices that encourage the presence of beneficial microbes are discussed further in the following ATTRA publications that may be found at https://attra.ncat.org/topics/soils-compost/:
• Sustainable Soil Management
• Drought Resistant Soil
• Overview of Cover Crops and Green Manures
• Managing Soils for Water: How Five Principles of Soil Health Support Water Infiltration and Storage
Omar and Rex can be contacted via email (omarr@ncat.org, rexd@ncat.org) or via phone (530) 530-7338.